Instrumental controls describe the controls necessary to properly set up the flow cytometer. This is done using negative controls to adjust voltages and create gating strategies.
-
Flow cytometry beads:
There are a few variations on beads/particles that are useful in setting parameters on the flow cytometer and making sure the cytometer is working properly.
- Quality control beads: Used to verify that the flow cytometer is functioning properly within vendor/facility specifications ensuring peak performance. These beads provide confidence that cytometer settings are consistent across experiments, increasing reproducibility of data generation.
- Compensation beads: Used to determine spillover from one fluorescent channel into another in a multicolor experiment. Compensation beads are useful when experimental cells are extremely limited, and there are not enough to be used as single fluorophore controls. (More information is provided in the Single fluorophore controls section below.)
- Counting beads: Used when determining absolute cell number is important. These beads are mixed into the sample, and calculations are performed to determine cell number or concentration.
-
Unstained sample:
Unstained samples are processed exactly like the experimental samples (same washes and incubation steps) but without the addition of antibodies or fluorescent markers. These samples can be used to identify cell populations of interest by differentiating single cells from debris and doublets or cell clumps. Unstained samples also provide information about autofluorescence in the sample. Autofluorescence is the background fluorescence level in the sample being examined.
Different cells and conditions have different levels of autofluorescence. For example, samples that are fixed and permeabilized often have increased levels of background fluorescence compared to live cell samples. Using unstained samples, optimal photomultiplier tube voltages can be determined and set for specific cell types/conditions.
-
Isotype control:
Isotype controls are antibodies that match the experimental antibodies in all characteristics except that the target antigen for the isotype control antibody is not present in the sample being investigated. This means that the isotype control antibody should have been developed from the same species, have the same reactivity, be used at the same concentration, and, if applicable, be conjugated to the same fluorophore as the experimental antibody. The isotype control sample provides information about nonspecific antibody binding in the sample.
This nonspecific binding could be a result of the antibody binding to Fc receptors or other cellular components in the sample. The isotype control helps to distinguish true binding from artifact.
Antibodies to epitope tags can work as isotype controls for samples that do not express the specific tag. In the example below a rabbit monoclonal antibody, V5 Tag Recombinant Monoclonal Antibody, was used as an isotype control for a rabbit monoclonal antibody of interest. This is a good isotype control because both antibodies were generated using the same processes (host: rabbit, isotype: IgG, reactivity: human, clonality: recombinant monoclonal).
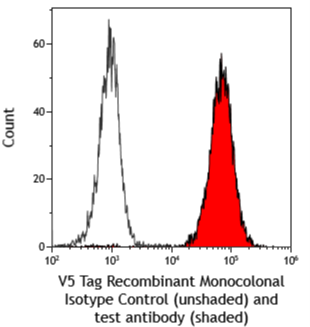
Figure 1. Example isotype control data comparing a tag recombinant monoclonal antibody (unshaded histogram) being used as an isotype control for a recombinant monoclonal test antibody (red shaded histogram).
-
Secondary antibody-only control:
Secondary antibody-only controls are used when indirect staining is performed. In these experiments, a primary unconjugated antibody is detected by a fluorescently labeled secondary antibody. The secondary antibody-only control does not contain any primary antibody, only the fluorescently labeled secondary antibody is added. This control is important for determining whether there is any nonspecific binding from the secondary antibody.
-
Single fluorophore controls:
Single fluorophore controls are used in multicolor flow cytometry experiments and are important controls used to calculate and apply compensation. Each fluorophore used for the multicolor flow experiment is added separately to individual samples. If the experiment uses FITC, PE, and APC, then FITC, PE, and APC would each be added to a separate sample creating a FITC-only control, a PE-only control, and an APC-only control. By having individual controls stained with each of the fluorophores separately, the spillover of each fluorophore into the other detectors can be determined and compensated. For example, FITC has substantial spillover into the PE channel which can result in a false double positive (FITC+PE+) population. After appropriate compensation is calculated and applied, this previously observed double positive population is seen as a FITC+ population.
-
Fluorescence Minus One (FMO) controls:
When multiple fluorophores are combined into a single sample, they can affect the spread of fluorescence into neighboring channels. FMO controls are therefore important for accurately setting gates and gating out spillover from another channel. The FMO controls are samples that include all of the fluorophores being used in the multicolor panel except for one. If the experiment uses FITC, PE, and APC, the FMO controls would be: FITC+PE+APC-, FITC+PE-APC+, and FITC-PE+APC+. These controls provide a more accurate gating strategy than using only unstained and single fluorophore controls.
Experimental controls describe the controls required to properly assess the presence of experimental targets and evaluate experimental questions. This is done using both negative and positive controls to determine differences in expression of targets and antibody binding.
The example below examines the expression of phosphorylated KAP-1. In this case, phosphorylation is induced by treating HEK293T cells with etoposide. It was important to compare untreated cells to etoposide-treated cells to validate specificity of the antibody and that the experimental conditions used were accurate.
Flow cytometry is a complex biological application that can generate a large amount of data for a single sample. The use of large multicolor panels is becoming increasingly common especially with advances in spectral flow cytometry. As the number of colors increases in a flow cytometry experiment, the experimental complexity increases exponentially making the use of appropriate controls critical for accurately collecting and deciphering the data generated. Accounting for both instrumental and experimental controls will increase success and reproducibility of flow cytometry experiments.